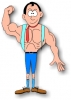
2021.06.03
認識「健康體能」民眾也許感到很疑惑,以往聽過「體適能」,現又有「健康體能」,二者究竟有何不同呢?「體適能」(Phyical fitness) 是國內體育界沿用已久的名詞,事實上包含兩大類,一為 「與運動競技有關的體適能」(Sport-related fitness),另一為「與健康有關的體適能」(Health-related fitness),本署關心的是與民眾健康有關的體適能,因此稱之為 「健康體能」。以後,並將沿用本名詞推展各相關業務,以促進民眾健康。 二、「健康體能」的涵意 所謂「健康體能」是指人的器官組織如心臟、肺臟、血管、肌肉等都能發揮正常功能,而使身體具有勝任日常工作、享受休閒娛樂及應付突發狀況的能力。 人的體能狀況可經由測量得知,且可利用規律活動或運動來改進,本署期待國民均有健康的身體及適當的體能,因此將利用本書介紹成年人評估健康體能的方法,並提出適當的運動建議。 三、健康體能的四大要素 (一) 心肺耐力 為健康體能四大要素中最重要的一項,其所涉及的範圍包括:心臟、肺臟、血管及血液等器官組織系統的機能。 運動生理學家和醫學研究者都已一致證實心血管循環耐力是體能評量的最重要指標。 (二) 肌力與肌耐力 肌耐力經常被人誤以為是肌肉力量,其實肌肉力量代表的是某一部位的肌肉或肌群一次能發揮的最大力量,而肌肉耐力則是某一部位肌肉或肌群在從事反覆收縮動作時的一種持久能力;或是指有關的肌肉維持某一固定用力狀態持久的時間而言。 肌力與肌耐力的訓練可以使肌肉纖維變粗,除了增加本身所能發出的力量外,也增強肌肉的耐久能力。身體任何大小的動作都是靠著肌肉牽引骨骼而完成的,肌肉本身若無法發出適當的力量,有些動作會顯得相當吃力或無法完成,也容易產生肌肉疲勞。很多成年人患有下背部疼痛,身體肌力不足是主要的原因之一。 (三) 柔軟度 這種能力代表的是人體的關節可以活動的最大範圍。而真正影響柔軟度的因素除了骨骼本身的結構外,還有肌肉、肌腱、韌帶、軟骨組織等。較佳的身體柔軟度表示肢體軀幹在運動、彎曲、伸展、扭轉時都比較輕鬆自如,同時也可以使肌肉與韌帶受到較好的保護而免於因受力而受傷。 (四) 身體組成(身體脂肪百分比) 從健康的觀點,肥胖是威脅生命的高危險因子,與心臟病、高血壓、膽囊疾病、糖尿病、肺活量減少、高脂血症及骨骼關節的疾病都有很密切的相關。因此身體脂肪百分比也是評估健康體能的重要項目,隨著文明的進步和生活水準的提昇,人類身體活動的機會減少,而熱量攝取常超越身體所需,使肥胖日漸增加。因此,要保持身體適當的脂肪百分比,除了需要均衡飲食外,更需要加強運動,藉以燃燒脂肪消耗身體過剩熱量,同時亦是達成體重控制的良好方法。 四、體能與健康的關係 現代社會以機器取代人力,因此一般工作與日常生活只需要普通的體能即可勝任,那麼,為什麼要提倡健康體能呢?事實上,一個人的體能狀況與生活品質和健康有密切的關係。 體能的衰退對個人有雙重影響,第一、年紀愈大,如果體能很差,常不足以應付日常活動之所需,因而無法獨立生活,生活品質低落,第二、體能衰退與缺乏運動息息相關,而缺乏運動又是危害健康的重要危險因子。 一般人的心肺耐力(以最大攝氧量代表),自25歲以後,每年約減少 1%,因此到了75歲時,心肺耐力可能只有年輕時的一半。此外,體脂肪由青年到中年迅速增加,肌肉量由中年至老年則迅速減少。 而缺乏運動者與經常運動者比較,罹患冠狀動脈心臟病的機會增加1.5至2.4倍,發生糖尿病的機會增加2至4倍,得到大腸癌的機會則增加2至5倍,據估計如果全民均從事適當運動,人口中因上述疾病而死亡的人可以減少三分之一。此外,缺乏運動與高血壓、高脂血、骨質疏鬆都有密切關係。 推廣健康體能活動有兩個好處,對個人而言,可提昇個人對自我體能狀況及適當運動重要性的認知,進而培養規律運動的習慣,以促進個人的健康體能,提高生活品質,並減少因缺乏運動而產生之退化性或慢性疾病的發生,延長健康的壽命。對社會而言,可有效提昇全民身體活動量,以促進國民健康體能狀況,進而提昇國家競爭力,節省醫療支出,減少中高年齡人口對社會及家庭的負擔。 能
2021.06.03
有關胃腸道的細菌性毒素之發表口服活性碳會吸附腸道內有毒物質, 本身不被人體吸收,過去是使用在緊急治療藥物或化學品中毒之病人。 近年來的研究發現活性碳會吸附干擾胃腸道的細菌性毒素、消化性毒素及其他有機性廢物、解除腸內滯留氣體及有關症狀。 還有實驗證明某些 特殊劑型的口服活性碳 (例如: 多孔分散吸附元、微球細粒吸附碳等), 可在腸胃道選擇性的吸附重金屬、indole及p-cresol,以減少人體吸收的機會,進而延緩慢性腎衰竭患者透析起始時間及改善尿毒症狀。
2021.04.02
學術分享 Blood omega-3 fatty acids and death from COVID-19: A pilot studyProstaglandins, Leukotrienes and Essential Fatty Acids Volume 166, March 2021, 102250 Short communication Blood omega-3 fatty acids and death from COVID-19: A pilot study Author links open overlay panelArashAsheraNathan L.TintlebcMichaelMyersdLauraLockshonaHeribertBacarezaeWilliam S.Harrisbf a Samuel Oschin Comprehensive Cancer Institute at Cedars-Sinai Medical Center, Los Angeles, CA b Fatty Acid Research Institute, Sioux Falls, SD c Department of Mathematics and Statistics, Dordt University, Sioux Center, IA d Los Alamitos Medical Center, Los Alamitos, CA e Department of Medical Affairs, Cedars-Sinai Medical Center, Los Angeles, CA f Department of Internal Medicine, Sanford School of Medicine, University of South Dakota, Sioux Falls, SD Received 6 January 2021, Revised 17 January 2021, Accepted 17 January 2021, Available online 20 January 2021. Show less Add to Mendeley Share Cite https://doi.org/10.1016/j.plefa.2021.102250Get rights and content Highlights • Nutrition-based interventions to reduce COVID-19 morbidity/mortality are needed. • The Omega-3 Index (O3I) was measured in banked blood from 100 COVID-19 patients. • Risk for death was analyzed as a function of quartiles (Q) of the O3I. • Patients in Q4 (O3I ≥ 5.7%) vs. Q1–3 were 75% less likely to die (p = 0.07). • These pilot data suggest that a higher O3I may lower risk for death from COVID-19. Abstract Very-long chain omega-3 fatty acids (EPA and DHA) have anti-inflammatory properties that may help reduce morbidity and mortality from COVID-19 infection. We conducted a pilot study in 100 patients to test the hypothesis that RBC EPA+DHA levels (the Omega-3 Index, O3I) would be inversely associated with risk for death by analyzing the O3I in banked blood samples drawn at hospital admission. Fourteen patients died, one of 25 in quartile 4 (Q4) (O3I ≥5.7%) and 13 of 75 in Q1–3. After adjusting for age and sex, the odds ratio for death in patients with an O3I in Q4 vs Q1–3 was 0.25, p = 0.07. Although not meeting the classical criteria for statistical significance, this strong trend suggests that a relationship may indeed exist, but more well-powered studies are clearly needed. Previous article in issue Next article in issue Keywords Omega-3 fatty acids Eicosapentaenoic acid Docosahexaenoic acid COVID-19 Total mortality Omega-3 index Inflammation 1. Introduction COVID-19, the illness caused by the severe acute respiratory syndrome coronavirus 2 (SARS-CoV-2), has been diagnosed in over 80 million people worldwide as of the end of 2020, and over 1.8 million have died [1]. Although 81% of symptomatic individuals have relatively mild disease, 14% will develop severe disease characterized by dyspnea, hypoxia, or >50 percent lung involvement by imaging, with the remaining 5% developing critical disease characterized by respiratory failure, shock, and/or multiorgan dysfunction [2]. Severe and critical disease from COVID-19 is associated with advancing age (especially over 65 years), male gender, chronic lung disease, obesity, cardiovascular disease including hypertension, diabetes, and other chronic medical conditions. Severe COVID-19 disease and death is, in part, mediated by rapid elevations of inflammatory cytokines including TNF-alpha, IL-1β, and IL-6 leading to a cytokine release syndrome or “cytokine storm.” [3] Accordingly, an attractive preventative approach to COVID-19 infection is to minimize cytokine release. Very long-chain omega-3 s (DHA and EPA) found in fish oils have a plethora of biological activities including directly and indirectly modulating inflammatory responses and cytokine release [4], [5], [6]. In non-COVID-19 ettings, higher intakes [7], [8] and blood levels [9], [10] of these omega-3 s are associated with lower levels of circulating inflammatory cytokines, and intervention with fish oils reduces levels [11], [12]. EPA and DHA are precursors to a suite of inflammation-resolving mediators (IRMs; resolvins, maresins and protectins [13]) that actively regulate the resolution of acute inflammation. IRMs down-regulate cytokine production and promote a return to homeostasis via monocyte/macrophage-mediated uptake of debris, apoptosis of neutrophils, and clearing of microbes. Accordingly, higher intakes of EPA and DHA (which result in higher RBC EPA+DHA levels, hereafter called the Omega-3 Index, O3I [14], [15]) have been proposed to lower the risk for adverse outcomes from COVID-19 [16], [17], [18], [19], [20], [21], [22], and case reports suggesting benefit have been published [23], [24]. Given the profound public health concerns related to the current COVID-19 pandemic, modifiable risk factors for developing severe and critical complications are urgently needed, especially ones that may be easily implemented and nutritionally based. Despite the known mechanisms by which IRMs and omega-3 fatty acids support the active, endogenous resolution of inflammatory mechanisms, to our knowledge no study has explored the relationship between omega-3 tissue levels and COVID-19 outcomes. The primary objective of this pilot study was to define the relationship between O3I and death from COVID-19. We hypothesize that a higher O3I is associated with lower risk for death in these patients. 2. Methods 2.1. Subjects We studied 100 patients hospitalized at Cedars-Sinai Medical Center from March 1, 2020 onwards with confirmed COVID-19 infection who met the criteria for inclusion, which were the availability of basic demographic data, clinical outcomes and an EDTA blood sample (drawn within 10 days of diagnosis) stored at −80 °C at the Cedars-Sinai biorepository. If more than one sample was available, the first sample drawn after the time of diagnosis was utilized. We utilized sample remnant protocol Pro00036514 to obtain blood samples for this study. Briefly, a protocol was submitted to the Enterprise Information Service (EIS) team and staff members of the Biobank. After review and approval, the EIS/Biobank team pulled the samples and the data, de-identifying each sample by assigning a study-specific number. Using this protocol, we obtained a limited data and blood sample set to conduct this pilot investigation. The study was approved by the IRB of Cedars-Sinai Medical Center (STUDY-00,000,779). 2.2. Clinical outcomes The primary outcome was death from COVID-19 infection. 2.3. Laboratory Blood samples were thawed at the biorepository and one drop was placed on a dried blood spot collection card pre-treated with antioxidants to protect the fatty acids from degradation. The cards were then shipped overnight in batch to OmegaQuant Analytics (Sioux Falls, SD) for analysis of fatty acids and calculation of the Omega-3 Index [25]. Briefly, blood spots were transferred to a reaction vial and FA methyl esters (FAMEs) were generated using boron trifluoride in methanol by heating for 45 min at 100 °C. FAMEs were extracted into hexane (after the addition of water) and analyzed using a GC2010 Gas Chromatograph (Shimadzu Corporation, Columbia, MD) equipped with a SP2560, 100-m column (Supelco, Bellefonte, PA). FAMEs were identified by comparison with a standard mixture (GLC, Nucheck Prep, Elysian, MN). Analysis was conducted using an internal-standard-based, three-point calibration curve to quantify levels of 24 FAMEs which were each expressed as a percent of total FAs. The O3I was calculated from the dried blood spot EPA+DHA value as described previously [25]. The analytical coefficient of variation for the O3I is <5%. 2.4. Statistical methods The study sample is described using standard descriptive statistics (means and standard deviations, medians and ranges, and counts and percentages). Additional descriptive summary of the sample is provided by stratifying using quartiles of the O3I or by comparing Q4 to Q1–3. The distribution of age, sex and do not resuscitate (DNR) status by O3I quartiles or top 25%/bottom 75% was tested using an F-test (age) or Chi-squared test (sex, DNR status). The primary analysis examined the hypothesis that O3I is associated with risk for death. We first predicted death using unadjusted logistic regression models by quartiles of the O3I using a Chi-squared test to evaluate overall (4-group) association. We also predicted death by age, sex and DNR status to evaluate and confirm known associations between risk for death and these factors. Subsequently, significant association between O3I quartiles and death was tested using logistic regression and by comparing Q4 with Q1–3 in both unadjusted models and models adjusted for age and sex. To account for the small sample sizes present in this study, primary model results use Firth's adjustment for small sample sizes in logistic regression using the logistf package in R [26]. We also report conventional logistic regression F-test results for comparison. A 2-tailed p-value of 0.05 was used for statistical significance. 3. Results 3.1. Sample description The study sample is described in Table 1. They were predominantly men, the mean age was over 70, and they were admitted between April and July 2020. Nearly 40% were under a DNR order instituted at some point during the hospitalization, and 14% died during hospitalization. The average O3I was 5.09%, and the median was 4.75%. Table 1. Descriptive statistics. Characteristics of sample % (X/N) or Mean (SD; min,max) Sex – Male 59% (59/100) Age 72.5 (16.5; 25,100) Month of data collection April – 47% (47/100) May – 25% (25/100) June – 15% (15/100) July – 13% (13/100) Red blood cell EPA+DHA (% of RBC fatty acids; the Omega-3 Index, O3I) 5.09% (1.62%; 2.87%, 13.79%) Died 14% (14/100) DNR orders 38% (38/100) Table 2 illustrates the association of the O3I by age, sex and do not resuscitate (DNR) status. Higher O3I values were significantly related to older age, with the highest mean age in the third quartile (79.8 years). Men and those who were not DNR tended to have higher O3I values, but these trends were not statistically significant. Table 2. Demographic profile of participants by category of the O3I. Categorical by O3I Quartile Age Mean (SD) Sex - Male% (x/n) DNR% (x/n) Q1 (O3I<4.0%) 63.0 (18.9) 64% (16/25) 44% (11/25) Q2 (4.0%<O3I<4.7%) 71.0 (16.1) 56% (14/25) 28% (7/25) Q3 (4.7<O3I<5.7%) 79.8 (13.3) 52% (13/25) 52% (13/25) Q4 (O3I≥5.7%) 76.3 (12.7) 64% (16/25) 28% (7/25) P-valuea 0.0014 0.77 0.20 Comparing O3I Q4 vs Q1–3 Q1–3: O3I<5.7% 71.3 (17.5) 57.3% (43/75) 41.3% (31/75) Q4: O3I≥5.7% 76.3 (12.7) 64% (16/25) 28% (7/25) P-valuea 0.19 0.56 0.23 a F-test (age and O3I) or Chi-square test (sex, DNR and O3I). 3.2. Unadjusted analyses Relationships between omega-3 status and fatal outcomes are shown in Table 3. As expected, older patients and those under a DNR order were more likely to die. In O3I Q4, there was one death (a 66-year-old male under a DNR order), whereas there were 13 deaths among the 75 patients in Q1–3. In the unadjusted model (and focusing on the small-sample size p-values), there was a significant difference in risk for death across quartiles of the O3I (p = 0.047), with those in the highest O3I quartile (Q4) having an odds ratio (OR) of 0.39 (p = 0.34) relative to Q1. In an unadjusted comparison between Q4 and Q1–3, the OR for death in Q4 was 0.28 (p = 0.11) or 3.6-fold higher (1/0.28) higher risk in Q1–3 vs. Q4. For comparison, the risk for death from COVID-19 associated with being 1-decade older was about 1.3-fold higher (p = 0.14). Hence, a low O3I appeared to be at least as predictive of risk for death as being 10-years older. The higher OR for death in Q3 was largely accounted for by age as the mean age in Q3 was the highest of all. The OR in Q3 was markedly attenuated in the adjusted analysis (Table 4). Table 3. Unadjusted associations of the Omega-3 Index and demographic variables with death. Risk factor Death% (x/N) Unadjusted Models OR (95% CI)a Firth's test P-valuea F-test P-valueb Female 14.6% (6/41) 1.00 Male 13.6% (8/59) 0.90 (0.30, 2.83) 0.85 0.88 Age Per decade – 1.33 (0.92, 2.08) 0.14 0.13 DNR Yes 34.2% (13/38) 21.76 (4.90, 206.44) <0.0001 0.001 No 1.6% (1/62) Categorical by O3I Quartile Overall model F-test 0.047 0.03 Q1 (O3I<4.0%) 12.0% (3/25) 1.00 Q2 (4.0%<O3I<4.7%) 8.0% (2/25) 0.68 (0.11, 3.86) 0.66 0.64 Q3 (4.7<O3I<5.7%) 32.0% (8/25) 3.13 (0.82, 14.30) 0.10 0.10 Q4 (O3I≥5.7%) 4.0% (1/25) 0.39 (0.04, 2.61) 0.34 0.32 Comparing O3I Q4 vs Q1–3 Q1–3: O3I<5.7% 17.3% (13/75) 1.00 Q4: O3I≥5.7% 4.0% (1/25) 0.28 (0.03, 1.26) 0.11 0.13 Bold and italics is used for p-values less than the significance level of 0.05. a Using the Firth's modified score procedure for small sample sizes. b Standard F-test from Logistic Regression ignoring small sample sizes. Table 4. Associations of the omega-3 index with death adjusted for age and sex. Risk factor Adjusted Models OR (95% CI)a Firth's test P-valuea F-test P-valueb Categorical by O3I Quartile Overall model F-test 0.078 0.0499 Q1 (O3I<4.0%) 1.00 Q2 (4.0%<O3I<4.7%) 0.58 (0.09, 3.35) 0.54 0.52 Q3 (4.7<O3I<5.7%) 2.18 (0.52, 10.80) 0.29 0.28 Q4 (O3I≥5.7%) 0.30 (0.03, 2.08) 0.22 0.22 Comparing O3I Q4 vs Q1–3 Q1–3: O3I<5.7% 1.00 Q4: O3I≥5.7% 0.25 (0.03, 1.11) 0.071 0.099 Bold and italics is used for p-values less than the significance level of 0.05. a Using the Firth's modified score procedure for small sample sizes. b Standard F-test from Logistic Regression ignoring small sample sizes. 3.3. Adjusted analyses In models adjusted for age and sex (Table 4), the overall relationship across quartiles became somewhat weaker (i.e., from p = 0.047 to 0.078), although the OR at Q4 (vs Q1) decreased slightly (0.39 to 0.30; p-value also decreased somewhat from 0.34 to 0.22). Comparing Q4 to Q1–3, the OR decreased slightly (from 0.28 to 0.25), and the p-value also decreased from 0.11 to 0.07. An OR of 0.25 implies that risk for death in COVID-19 patients with an O3I >5.7% was ~75% less than it was for patients with an O3I<5.7%. 4. Discussion The 2020 COVID-19 pandemic has had devasting effects on morbidity and mortality worldwide. While vaccines may soon slow the spread and drugs may help diminish the adverse effects of infection, preventative measures – ideally ones that are cheap, safe, and readily accessed by large numbers of people – that modulate the most severe disease outcomes are desperately needed. Some evidence is accumulating for a potential benefit of vitamin D [27], and here we examined another nutrient, omega-3 fatty acids, which like vitamin D, have multiple anti-inflammatory effects and may also reduce risk for adverse COVID-19 outcomes. In this pilot study we compared the associations between the O3I and risk for death from COVID-19 in 100 patients. We found (in age and sex-adjusted models) that those patients with an O3I at 5.7% or greater were at about 75% lower risk for death compared with those below that value (p = 0.071). Although not meeting the criteria for classical statistical significance, this strong trend suggests that a relationship may indeed exist, but more well-powered studies are clearly needed. The OR for death was higher in Q3 than in the other quartiles including Q4. Part of the reason for this was the mean age (and the percent of patients with DNR orders) was the highest in Q3. Accordingly, in the age-adjusted model the OR in Q3 dropped by a third. The comparison of Q4 to Q1–3 has historical precents. For example, in the Framingham Heart Study, significantly larger brain volumes and better cognitive function were observed in Q4 vs Q1–3 [28], and 10-year risk for dementia was 47% lower in subjects with omega-3 levels in Q4 vs Q1-Q3 [29]. More generally, higher omega-3 FA blood levels have been associated with the better clinical outcomes for cardiovascular disease [30,31], post-MI cardiac remodeling [32], telomere attrition [33], attempted suicide [34] and total mortality [35]. These data suggest that in some settings there may be threshold effects of the O3I. This study used the O3I as a biomarker of omega-3 FA status. This RBC-based metric has advantages, particularly in the acute hospitalization setting. This is because, much like a hemoglobin A1C versus plasma glucose, the O3I is a better long-term reflection of tissue omega-3 levels versus plasma omega-3 levels [36], and it would thus be less affected by an acute change in omega-3 intake, as might happen with hospitalization for an acute illness [37]. The O3I has been validated [25] and used in several interventional [14] and prospective cohort studies such as the Framingham Heart Study [31] and the Women's Health Initiative Memory Study [38]. The O3I is also easily modified by increasing the intake of oily fish (e.g., salmon, herring, mackerel, albacore tuna, etc.) which are rich in EPA and DHA, and dietary supplements of omega-3 will also raise levels [15]. The average O3I in this study was 5.1% which is similar to that seen other US-based studies [39], [40]. We also examined the associations between risk for death and EPA and DHA individually and with the omega-6:omega-3 ratio. We found the same directional relationships as with the O3I but they were not as statistically strong (data not shown). Multiple randomized clinical trials (RCTs) are currently (as of January 2021) underway testing the hypothesis that treatment with omega-3 fatty acids (EPA and DHA) will have beneficial effects on a variety of aspects of COVID-19 infection. Although their outcomes are not yet known, there are compelling scientific reasons to expect that these studies will be positive (and these same reasons formed the foundation for the present study). The data supporting a possible beneficial role for omega-3 fatty acids in COVID-19 infection come from past epidemiological, interventional, therapeutic, and basic science studies. For example, in the Framingham Offspring study [41], the O3I was inversely associated with 10 separate inflammatory biomarkers (e.g., CRP, IL-6, ICAM-1, LpPLA2, TNF receptor 2, and osteoprotegerin). In intervention studies [11], EPA supplementation alone (3 g/d for 10 weeks) significantly reduced the expression of TNFα from LPS-stimulated monocytes as did a similar dose of DHA which, in addition, lowered IL-6 and MCP-1. EPA+DHA supplements had similar effects [42]. Meta-analyses of multiple RCTs confirmed that treatment with omega-3 fatty acids routinely lowers cytokine levels [43], [44], [45]. More important than studies of effects on intermediate markers are clinical findings from RCTs. Langlois et al. [5] summarized the results of 12 RCTs of omega-3 treatment in 1280 intensive-care-unit patients with acute respiratory distress syndrome. There was a significant improvement in measures of blood oxygenation in the treated patients and strong trends (p ≤ 0.08) for reduced ICU length of stay and duration of mechanical ventilation. Overall mortality, hospital length of stay and infectious complications were unaffected. As noted earlier, the potential mechanisms underlying these actions are multiple. EPA/DHA are substrates for the production of IRMs which cannot be made if the parent compounds are not present. Examples of some of the functions of IRMs were summarized by Calder as, “Resolvin E1, resolvin D1 and protectin D1 all inhibit trans-endothelial migration of neutrophils, so preventing the infiltration of neutrophils into sites of inflammation; resolvin D1 inhibits IL-1β production and protectin D1 inhibits TNF-α and IL-1β production.” [46] Higher EPA/DHA levels reduce arachidonic acid (the omega-6 cousin of EPA) membrane levels [47] for the production of some pro-inflammatory oxylipins (certain prostaglandins and leukotrienes). Quite independently of the synthesis of these mediators, the presence of EPA/DHA in inflammatory cells blocks the activation of the key pro-inflammatory transcription factor, nuclear factor kappa B thus retarding the entire intracellular inflammatory cascade [48,49]. This effect is downstream from the action of these fatty acids on membrane G-protein coupled receptors GPR40 and GPR120 and nuclear peroxisome proliferator-activated receptors (reviewed in [50]). Finally, EPA/DHA insert into cell membrane phospholipids and disrupts lipid rafts so as to disassemble surface receptors thereby blocking incoming inflammatory signals [51]. All of these actions together result in a muted “cytokine storm” which, in alveolar macrophages and elsewhere, can result in death from COVID-19 [3]. 4.1. Limitations Given the pilot nature of this exploratory study, a number of limitations are acknowledged. Firstly, the sample size was small and further studies are needed to replicate this finding and to explore potential risk thresholds of the O3I. Secondly, the limited resources and resulting access to the full electronic medical record for more detailed chart review significantly reduced the amount of potentially relevant information on comorbidities and other demographic data besides age and sex (e.g., BMI). Although data on maximal interventions applied during hospitalization were available, the reasons why any given patient was administered a given treatment are not known and could obviously have been influenced by external factors (e.g., ventilator availability) or internal factors (e.g., a DNR order). Accordingly, such information was deemed to be of little utility in this pilot study. The population of patients in this study had an O3I that was typical of the US[39], which unfortunately means that levels were generally low. Future studies should endeavor to include patients with a wider range of O3I to examine these relationships more clearly. 4.2. Conclusions Given the profound public health concerns related to the current COVID-19 pandemic, modifiable risk factors for developing severe and critical complications are urgently needed. Despite the known mechanisms by which IRMs and omega-3 fatty acids support the active, endogenous resolution of inflammatory mechanisms, to our knowledge this is the first study that has explored the relationship between omega 3 tissue levels and the most severe COVID-19 outcome, death. Larger studies are urgently needed to confirm these findings. If an association is confirmed with a larger sample size, then this would lay the groundwork for testing the effects of increased oily fish intake and/or an inexpensive, safe, and widely available dietary supplement (DHA/EPA capsules) to optimize outcomes during this public health crisis. Author disclosure statements WSH holds an interest in OmegaQuant Analytics, LLC; and is a member of the Schiff Science and Innovation Advisory Board. The other authors have no conflicts of interest to disclose. Data availability statement The data that support the findings of this study are available from the corresponding author upon reasonable request. Funding statement This study was supported in part by Cedars-Sinai Medical Center (through the Cancer Clinical Trials Office), by the Fatty Acid Research Institute (for biostatistical support), and by a donation from Michael Myers (for blood analysis). CRediT authorship contribution statement Arash Asher: Conceptualization, Investigation, Methodology, Project administration, Supervision, Writing - original draft. Nathan L. Tintle: Formal analysis, Writing - review & editing. Michael Myers: Conceptualization, Resources, Methodology, Writing - review & editing. Laura Lockshon: Project administration, Writing - review & editing. Heribert Bacareza: Project administration, Writing - review & editing. William S. Harris: Conceptualization, Methodology, Resources, Writing - original draft.
2021.03.07
學術分享 The effect of omega-3 fatty acid supplementation on clinical and biochemical parameters of critically ill patients with COVID-19: a randomized clinical trialPublished: 29 March 2021 The effect of omega-3 fatty acid supplementation on clinical and biochemical parameters of critically ill patients with COVID-19: a randomized clinical trial Saeid Doaei, Somayeh Gholami, Samira Rastgoo, Maryam Gholamalizadeh, Fatemeh Bourbour, Seyedeh Elaheh Bagheri, Forough Samipoor, Mohammad Esmail Akbari, Mahdi Shadnoush, Fereshteh Ghorat, Seyed Alireza Mosavi Jarrahi, Narjes Ashouri Mirsadeghi, Azadeh Hajipour, Parvin Joola, Alireza Moslem & Mark O. Goodarzi Journal of Translational Medicine volume 19, Article number: 128 (2021) Cite this article 11k Accesses 290 Altmetric Metricsdetails Abstract Background Omega-3 polyunsaturated fatty acids (n3-PUFAs) may exert beneficial effects on the immune system of patients with viral infections. This paper aimed to examine the effect of n3-PUFA supplementation on inflammatory and biochemical markers in critically ill patients with COVID-19. Methods A double-blind, randomized clinical trial study was conducted on 128 critically ill patients infected with COVID-19 who were randomly assigned to the intervention (fortified formula with n3-PUFA) (n = 42) and control (n = 86) groups. Data on 1 month survival rate, blood glucose, sodium (Na), potassium (K), blood urea nitrogen (BUN), creatinine (Cr), albumin, hematocrit (HCT), calcium (Ca), phosphorus (P), mean arterial pressure (MAP), O2 saturation (O2sat), arterial pH, partial pressure of oxygen (PO2), partial pressure of carbon dioxide (PCO2), bicarbonate (HCO3), base excess (Be), white blood cells (WBCs), Glasgow Coma Scale (GCS), hemoglobin (Hb), platelet (Plt), and the partial thromboplastin time (PTT) were collected at baseline and after 14 days of the intervention. Results The intervention group had significantly higher 1-month survival rate and higher levels of arterial pH, HCO3, and Be and lower levels of BUN, Cr, and K compared with the control group after intervention (all P < 0.05). There were no significant differences between blood glucose, Na, HCT, Ca, P, MAP, O2sat, PO2, PCO2, WBCs, GCS, Hb, Plt, PTT, and albumin between two groups. Conclusion Omega-3 supplementation improved the levels of several parameters of respiratory and renal function in critically ill patients with COVID-19. Further clinical studies are warranted. Trial registry Name of the registry: This study was registered in the Iranian Registry of Clinical Trials (IRCT); Trial registration number: IRCT20151226025699N3; Date of registration: 2020.5.20; URL of trial registry record: https://en.irct.ir/trial/48213 Introduction In recent years, coronavirus infections have been a significant source of morbidity and mortality around the world [1]. Novel coronavirus 2019 (nCoV-2019), now known as acute respiratory syndrome coronavirus 2 (SARS-CoV-2), is responsible for the pandemic of coronavirus disease COVID-19 [2]. This infection usually causes a defined pattern of metabolic and clinical changes in affected patients [3]. Leukocytopenia, lymphopenia, and elevated C-reactive protein (CRP) can occur in the primary form of COVID-19. With disease progression, increasing levels of leukocytes, creatine kinase, and creatinine may also occur [4]. Host nutritional status has an important role in defense against viral infections [5]. Numerous studies have reported that malnourished humans are more susceptible to a wide variety of infections [6]. Indeed, proper nutrition can preserve the immune system and improve its function [7]. Nutritional deficiencies influence both immune response and viral pathogenic functions [5, 8]. Moreover, they may lead to oxidative stress in the host which can alter the genome of the virus, so that a normally benign or mildly pathogenic virus can convert to a highly virulent pathogen [6]. Omega-3 polyunsaturated fatty acids (n3-PUFAs) are important mediators of inflammation and acquired immune responses and can amplify anti-inflammatory responses [9]. Recent studies have been shown that n3-PUFAs including eicosapentaenoic acid (EPA), docosahexaenoic acid (DHA), and α-linoleic acid (ALA) can increase the stability of the cell membrane, regulate immune function, block hyper inflammatory reactions, and reduce the incidence of systemic inflammatory response syndrome (SIRS), multiple organ dysfunction syndrome (MODS), and complications of infection [10]. Chen et al. reported a lower mortality rate in septic patients who received total parenteral nutrition containing 100 ml (ml) n3-PUFAs daily for 4 weeks. Also, the treatment group had a higher ratio of T helper to inducer lymphocytes and CD4 to CD8 lymphocytes in comparison with the control group during the first 7 days of follow-up [11]. Pluta et al. found that patients with chronic kidney disease, who daily received one capsule of omega 3 including 1000 mg n3-PUFAs containing 330 mg of EPA, 220 mg of DHA, and 100 mg of other acids such as alpha-linolenic acid (ALA) had lower urinary excretion of monocyte chemoattractant protein-1 (MCP-1) after 6 months of follow-up. However, white blood cell (WBC) count and serum levels of CRP and MCP-1 did not change significantly [12]. Soleimani et al. reported that patients with diabetic nephropathy, who received 1 g omega-3 acids daily had higher circulating nitric oxide (NO) after 12 weeks of follow-up with no change in serum concentrations of high sensitivity (hs)-CRP or total antioxidant capacity (TAC) [13]. Coghill et al. demonstrated that human immunodeficiency virus (HIV) and human herpesvirus-8 (HHV-8) co-infected patients, who received 3 gr of omega-3 fatty acids daily (each pill contained about 230 mg EPA and 150 mg DHA), had lower serum concentrations of the inflammatory cytokine interleukin-6 (IL-6) and higher CD8 + counts at 12 weeks [14]. Omar et al. in a recent study found that end-stage renal disease patients on chronic hemodialysis, who daily received two grams of omega-3 (containing 360 mg EPA and 240 mg DHA) had lower serum levels of IL-6 and CRP at 3 months [15]. On the other hand, Rice et al. conducted a randomized, double-blind, placebo-controlled, multicenter trial and reported that the n-3 supplementation did not improve the primary end point of ventilator-free days or other clinical outcomes in patients with acute lung injury and may be harmful. Patients receiving the n-3 supplement had fewer ventilator-free days, intensive care unit–free days, and nonpulmonary organ failure–free days [16] Given suggestive evidence of the beneficial effects of n3-PUFAs on the immune system and the contradictory results of recent studies concerning the effects of omega-3 fatty acids in patients with viral infections, we aimed to examine the effect of omega-3 supplementation on inflammatory and biochemical markers in critically ill patients with COVID-19. Methods Study design and population This study was a double-blind, randomized clinical trial study carried out from May to July 2020 in critically ill patients infected with COVID-19 in Razi Hospital, Rasht, Iran. The inclusion criteria were the age between 35 and 85 years, diagnosis of COVID-19 confirmed by a positive RT-PCR nasopharyngeal swab, as well as symptoms such as severe pneumonia, fever, fatigue, dry cough, respiratory distress, and indicated for enteral nutrition. One hundred thirty five patients were assessed for eligibility and 128 patients met the inclusion criteria. Sample size was calculated using α = 0.95, β = 20%, ratio of unexposed to exposed of 2:1, and power of 0.8. An unequal randomization ratio (2:1) was used because of a fixed and limited budget for this research project. Therefore, more participants were randomized to the cheaper arm in order to facilitate greater overall recruitment in the face of a possibly high drop-out rate. The exclusion criteria included history of cardiovascular and/or lung diseases (n = 4), and diagnosis of malignant tumors (n = 1) because of their effects on current medical treatment and diet, consumption of omega-3 fatty acids duing the prior 3 months before the study (n = 1), history of hypersensitivity reactions to fish or its products (n = 1), did not complete the study because of death (n = 14) or no indication for enteral feeding any more (n = 13). The final analysis was performed on 101 critically ill patients infected with COVID-19 who were assigned to the intervention (n = 28) and control (n = 73) groups. Interventions This study was done in form of a double-blind trial. Though no placebo was used, the patients were not aware of their feeding contents, and the patients and researchers were not aware of the arms of the study. Finally, the results were analyzed by a person outside of the treatment team. The allocation to the groups was done through web-based randomization using https://www.randomizer.org. Sealed non-transparent envelopes with randomized sequences were used to hide the allocation. All participants received high protein formula as 30 kcal/kg/d through enteral feeding. The intervention group received one capsule of 1000 mg omega-3 daily (Vita Pharmed, Switzerland) containing 400 mg EPAs and 200 mg DHAs for 14 days through adding the supplement to their enteral formula. Omega-3 fatty acids fortified formula was administered to the case group by a nurse, who was not a member of the research team, for 2 weeks after the first 24 h of hospitalization in ICU. The omega-3 capsules were pierced with a syringe, then its contents were squeezed out into a prepared enteral formula, with thorough mixing. The control group received nutritional support including the isocaloric-isovolemic formula using the same route, except for the intervention. Data collection After collecting the written consent forms, the required information was collected using a pre-determined checklist including the following sub-scales: Anthropometric and medical history Data on age, weight, height, previous illnesses, medications, blood pressure, serum lipids, random blood glucose, and respiratory status was collected using medical records. Dietary intake Data on nutritional intake including caloric intake, type of formula, and dietary supplements were assessed using documents recorded in ICU sheets and patient logs. Biochemical and pathological indices Arterial blood gas (ABG) parameters [i.e., O2 saturation, arterial pH, partial pressure of oxygen (PO2), partial pressure of carbon dioxide (PCO2), bicarbonate (HCO3), and base excess (Be)], kidney function parameters [i.e., blood urea nitrogen (BUN), creatinine (Cr), and urine volume], as well as blood glucose, mean arterial pressure, white cell blood count (including neutrophil, lymphocyte, monocyte), the Acute Physiology and Chronic Health Evaluation II (APACHE II), Glasgow Coma Scale (GCS), hemoglobin (Hb), platelet (Plt), the partial thromboplastin time (PTT), serum electrolytes [i.e. sodium (Na), potassium (K), calcium, phosphorus (P)], albumin, and hematocrit (Hct) were measured at baseline and after 14 days of the intervention. These measurements were routinely performed in the hospital using standard kits and the required data were gathered from the lab test section of the ICU sheets. Statistical analyses Quantitative data were presented as the mean ± standard deviation and qualitative data were presented as the percentage and number to describe the status of demographic, social, and anthropometric measurements of the participants. The Kolmogorov–Smirnov test was used to verify the normal distribution. Independent T-test and chi-square test were used to compare results between groups at baseline. The core research question was whether the difference between groups of interest changed from the basal values to the values after treatment. The general linear model repeated measure was used to identify the group-by-time interaction effect of omega-3 supplementation on inflammatory and biochemical markers, comparing the values in intervention and control groups. Confounding factors including age, sex, body mass index (BMI), dietary intake, smoking, presence of background diseases such as diabetes and hypertension, and medication and supplements were adjusted for. Statistical analyses were performed using the SPPS software (version 20). P values less than 0.05 were considered statistically significant. Results Patient characteristics A total of 135 eligible patients were included in this study and randomization was done 1:2. Of these, seven patients were excluded based on the exclusion criteria (Fig. 1). In addition, another seven patients in the treatment group and twenty controls were excluded from analysis after randomization, because they died during the intervention period. Finally, 101 patients were included in the analysis, of which 28 were assigned to the treatment group and 73 were assigned to the control group. All baseline data in Table 1 were normally distributed. The groups were not significantly different in demographic or clinical characteristics at baseline, except for the lymphocyte count, which was lower in the intervention group (11.59 vs 13.26, P = 0.01). Fig. 1 Study flowchart Full size image Table 1 Baseline demographic and clinical data of the study groups Full size table Effects of omega-3 supplementation on 1-month survival rate The intervention group had significantly higher 1-month survival rate compared with the control group (21% vs 3%, P = 0.003). About 21% (n = 6) of the participants in the intervention group and only about 3% (n = 2) of the participants in the control group survived at least for 1 month after the beginning of the study. Effects of omega-3 supplementation on kidney function As summarized in Table 2, levels of BUN (35.17 vs 43.19, F = 4.76, P = 0.03) and Cr (1.29 vs 1.68, F = 5.90, P = 0.02) were significantly lower and the amount of urine excreted (2101 vs 1877.02, F = 12.26, p = 0.01) was significantly higher in the intervention group compared with the control group. Table 2 Comparison of clinical and biochemical parameters between the intervention group and control group after the intervention Full size table Effects of omega-3 supplementation on arterial blood gas (ABG) parameters After 14 days, the levels of arterial pH (7.30 vs 7.26, F = 19.11, P = 0.01), HCO3 (22.00 vs 18.17, F = 10.83, P = 0.01), and Be (−4.97 vs −3.59, F = 23.01, P = 0.01) were significantly higher in the intervention group compared with the control group. However, there were no significant differences among PO2 and PCO2 between two groups (Table 2). Effects of omega-3 supplementation on the Glasgow coma scale (GCS) On admission to the study, the mean GCS was 8.37 in the intervention group and 7.90 in the control group (P > 0.05). As shown in Table 2, it was significantly lower after 14 days of the study in both groups (7.90 vs 7.49, F = 6.07, P = 0.05). No significant difference was found in APACHE II score between the groups (15.54 ± 1.73 vs 15.42 ± 1.92., P = 0.78). Effects of omega-3 supplementation on serum electrolytes As shown in Table 2, the level of K was significantly reduced in the intervention group compared to the control group (4.00 vs 4.14, F = 10.15, P = 0.01) after 14 days. No significant differences were found between the levels of serum electrolytes including Na, Ca, and P in between two groups after intervention. Effects of omega-3 supplementation on blood clotting function and cell blood count (CBC) The lymphocyte count increased in the omega-3 group compared to the control group, though this effect was marginally significant (11.59 vs 11.80, F = 4.08, P = 0.05). There were no significant differences in levels of PTT, hematocrit, neutrophil, monocyte, hemoglobin, and Plt between the two groups (Table 2). Effects of omega-3 supplementation on the other blood factors As shown in Table 2, no significant differences were observed in the other factors including blood glucose, albumin, MAP, and O2 sat between two groups after intervention. Discussion To our knowledge, this is the first randomized clinical trial assessing the effect of omega-3 fatty acid supplementation in ICU patients with COVID-19. We found that administration of omega-3 PUFA significantly improved arterial PH, HCO3, and Be. This trial also supports our hypothesis that omega-3 supplementation can improve the level of indicators of kidney function including BUN, Cr, K, and urine volume. The results have indicated that omega-3 supplementation may increase the lymphocyte count and GCS. However, these increases were not statistically significant. In our study, omega-3 supplementation had a positive effect on 1-month survival rate of the critically ill patients with COVID-19. Many severely or critically ill COVID-19 patients have been reported to develop severe metabolic and respiratory acidosis [17, 18], indicating possible microcirculation dysfunction [18]. This study identified that omega-3 supplementation improved arterial PH, HCO3, and Be, which may be related to the effect of omega-3 supplementation on microcirculatory function. Several studies [19,20,21] have reported that omega-3 supplementation improved endothelial function and microcirculation. Vasil'ev et al. reported that therapy with omega-3 PUFAs increased tissue hemoperfusion, capillary blood flow, and tissue blood flow and generally improved microcirculation. Trevor et al. studied the effects of DHA and EPA supplementation on vascular reactivity and microcirculation. They found that DHA significantly enhanced vasodilator mechanisms, attenuated constrictor responses, and improved endothelium reactivity and microcirculation [22] It has been reported that renal abnormalities occurred in the majority of patients with COVID-19 [18, 23,24,25], and microemboli in renal vessels as a consequence of the prothrombotic state results in kidney damage. Furthermore, hypertension induced by pro-inflammatory status, increasing IFN-γ, IL-6, and IL-17 expression and CD8 + T cells in the kidney, angiotensin II-induced hypertension, and the endothelial dysfunction caused by the innate immune response seems to be involved in the kidney injury [26]. Renal involvement in COVID-19 was reported to be associated with higher mortality [18, 23,24,25]. Hence, it is crucial to identify adjuvant and protective therapies that improve kidney function in patients with severe COVID-19. The present study found that the administration of omega-3 PUFAs significantly decreased BUN, Cr, and K and increased urine volume in ICU patients infected with COVID-19. These findings suggest that omega-3 supplementation may be protective against progression to renal impairment, consistent with observations from animal models that showed that omega-3 PUFA supplementation reduces the progression of renal disease [27,28,29,30,31]. Moreover, studies in human subjects suggested that a higher dietary intake of PUFAs may improve creatinine clearance [32] and also may have a role in maintaining healthy kidney function [33]. In contrast, some studies have reported that omega-3 treatment did not protect renal function. Higashihara et al. assessed the effects of omega-3 PUFAs on kidney function in 41 patients with stages two or three chronic kidney disease (CKD); omega-3 supplementation did not alter kidney function, assessed using 24-h urine creatinine clearance. Another study demonstrated that omega-3 PUFA pre-treatment did not protect against renal function deterioration or ischemia-induced renal inflammation; however, tubular transport was improved [30]. Previous studies that have evaluated omega-3 PUFAs in the treatment of kidney disease have yielded contradictory results, which may reflect differences in study duration, dosage of omega-3, route of administration, and type of omega-3 fatty acids. Omega-3 PUFAs can change the lipid composition and cell function of lymphocytes and affect cellular immune function [10, 34, 35]. There is no consensus regarding the efficacy of omega-3 PUFAs on lymphocyte count. A meta-analysis of randomized control trials found that the value of postoperative lymphocyte count increased in response to omega-3 PUFAs supplementation [10]. Consistent with this finding, the results of the present study identified a marginal increase in lymphocyte count after omega-3 PUFAs supplementation. The results of the current study identified a significant difference in 1-month survival rate between two groups. Supplementation with omega-3 fatty acids may have immune-modulating [36,37,38] and organ-protective effects [39, 40]. Previous studies have reported that higher plasma levels of omega-3 PUFAs are associated with survival [41,42,43]. Moreover, a recent systematic review found that omega-3 supplements can improve survival in critically ill patients [44]. However, another recent review article concluded that there is weak evidence for beneficial effects of omega-3 supplementation on ICU or hospitalized survival in critically ill patients [45]. Heller et al. have reported that the effects and effect sizes of omega-3 on clinical outcome is dependent on the disease [46]. Our study had some limitations. Although the sample size was calculated with an acceptable power for the study, the results need to be confirmed in larger studies. Moreover, only one dosage of omega-3 was used, which makes it difficult to discuss the dose–response efficacy of the supplement. It is possible that we may have seen more benefit with a higher dose. Another limitation of this study was the short duration of the study. Furthermore, prognostic biochemical markers in COVID-19 including the inflammatory cytokines C-reactive protein (CRP), interleukin-6, and interleukin-10, were not measured due to limited resources. Another limitation of this study was that there was no access to an omega-3 fatty acid enriched formula, and therefore we had to add it manually. Conclusion In conclusion, this randomized, double-blind, clinical trial has shown that omega-3 supplementation has promising effects on acidosis and renal function and possibly can improve clinical outcomes of patients infected with COVID-19. Further clinical studies with different dosages of n-3 PUFAs, larger sample sizes, and longer duration are warranted. Availability of data and materials All data will be made available upon request. Abbreviations n3-PUFAs: Omega-3 polyunsaturated fatty acids Na: Sodium K: Potassium BUN: Blood urea nitrogen Cr: Creatinine HCT: Hematocrit Ca: Calcium P: Phosphorus MAP: Mean arterial pressure O2 sat: O2 saturation PCO2 : Partial pressure of oxygen WBC: White blood cells